Herzlich Willkommen
auf den Seiten des Deutschen Schwindel- und Gleichgewichtszentrums (DSGZ)
Wir möchten Ihnen auf diesen Web-Seiten unsere Arbeit in der Krankenversorgung und Forschung an der Ludwig-Maximilians-Universität München vorstellen, Ihnen die Kontaktaufnahme zu uns erleichtern und Sie über unsere Patientenangebote und Fortbildungsveranstaltungen informieren.
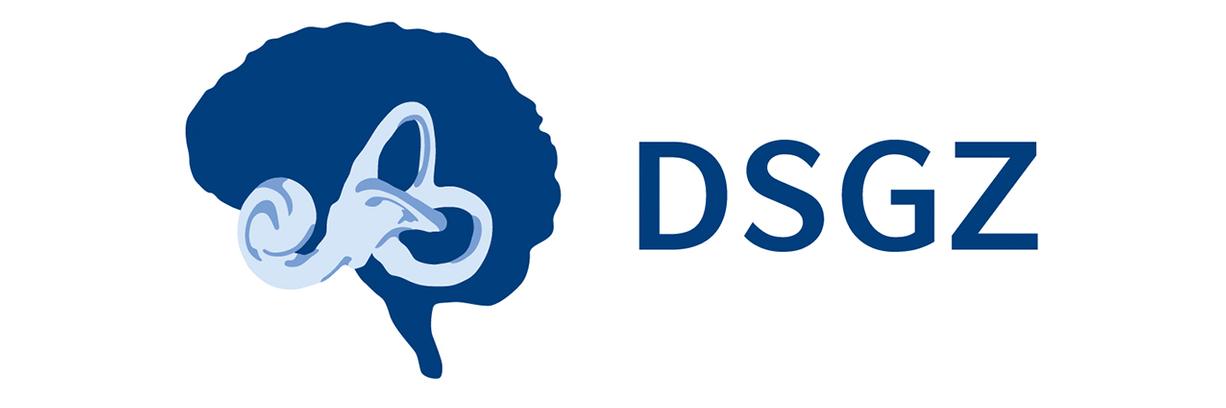
089 / 4400-76980
Montag - Donnerstag
9.30 - 12.30 Uhr und
13.30 - 15.00 Uhr
Freitag (optional)
9.30 - 12.30 Uhr
terminvereinbarung-ifb@med.uni-muenchen.de
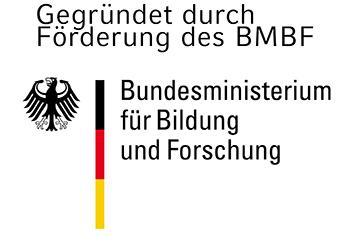
Spendenkonto
LMU-Klinikum
IBAN DE38 7005 0000 0002 0200 40
BIC BYLADEMMXXX
Unbedingt angeben: zu Gunsten DSGZ – Finanzstelle 80221002, Spende